Injection of Human Bone Marrow and Mononuclear Cell Extract into Infarcted Mouse Hearts Results in Functional Improvement
Abstract
Background:
We have previously shown that mouse whole bone marrow cell (BMC) extract results in improvement of cardiac function and decreases scar size in a mouse model of myocardial infarction (MI), in the absence of intact cells. It is not clear if these results are translatable to extracts from human BMC (hBMC) or mononuclear cells (hMNC), which would have significant clinical implications.
Methods:
Male C57BL/6J (10-12 weeks old) mice were included in this study. MI was created by permanent ligation of the left anterior descending artery. Animals were randomized into three groups to receive ultrasound-guided myocardial injections with either hBMCs extract (n=6), hMNCs extract (n=8) or control with 0.5% bovine serum albumin (BSA) (n=7). Cardiac function was assessed by echocardiography at baseline, 2 and 28 days post-MI. Infarct size and vascularity was assessed at 28 days post-MI.
Results:
hBMC and hMNC extract preserve cardiac function and result in smaller scar size post-MI when compared with the control group.
Conclusions:
The current study for the first time reports that hBMC and hMNC extracts improve cardiac function post-MI in a mouse MI model. Further studies are necessary to fully address the potential clinical benefits of these therapies.
INTRODUCTION
Cell therapy has emerged as a potential treatment option for cardiac ischemic disease. The benefits of this new approach have been tested in several clinical trials using bone-marrow-derived cells (BMCs) post myocardial infarction (MI) [1-6]. Growing evidence suggests that paracrine mechanisms, rather than cell differentiation or fusion, are responsible for the beneficial effects of cell therapy in improving cardiac function post-MI [7-12]. We have recently shown that intact mouse BMCs are not necessary to obtain benefits and that the intracellular components within mouse whole BMCs can reduce MI size and improve cardiac function when administered early following MI [12]. However, the impact of human BMC (hBMC) extract and human mononuclear cells (hMNC) extract on cardiac functional changes post-MI has not been investigated. Therefore, in this study, we take the first step towards clinical applicability by evaluating the efficacy of extracts obtained from hBMC and hMNC in a mouse model of MI.
MATERIAL AND METHODS
Male C57BL/6J (10-12 weeks old) mice were used for all experiments and were handled according to the guidelines of the Institutional Animal Care and Use Committee of the University of California, San Francisco.
MYOCARDIAL INFARCTION MODEL CREATION AND CARDIAC FUNCTION EVALUATION
MI was surgically-induced by permanent ligation of the mid left anterior descending coronary artery as previously described [12, 13]. Cardiac function was evaluated by echocardiography at baseline, 2 days post-MI (before injection), and at day 28 post-MI as previously described by our group [12-15]. Briefly, echocardiography was accomplished under isoflurane anesthesia (isoflurane 1.5%/O2 1.0 L/min) with the use of a Vevo 660 (VisualSonics, Toronto, Canada) equipped with a 30 MHz transducer. Two-dimensional echocardiography was used to determine left ventricle ejection fraction (LVEF) from end-diastolic and end-systolic volumes (EDV and ESV). Three cycles were measured for each assessment and the average values were obtained.
CELL AND EXTRACT PREPARATION
Fresh human whole BMCs were harvested from four young healthy males (mean age 26 ± 2 years). The MNCs fraction was selected using Ficoll gradient as previously described [16]. The concentration of the cells was adjusted to 105 viable cells/µl in phosphate-buffered saline containing 0.1% Tween 20, pH 7.4-20× OptiMax wash basic phosphate buffer saline (BPBS) with 0.5% bovine serum albumin (BSA). Cell-free extract was prepared by subjecting the cells to three freeze-thaw cycles using an ethanol/dry ice bath to lyse cells, followed by centrifugation at 14,000 rpm in a microcentrifuge to remove insoluble material. This resulted in an extract containing the intracellular soluble material.
ULTRASOUND-GUIDED INJECTIONS
After echocardiography on day 2 post-MI, animals were randomized into three groups to receive ultrasound-guided myocardial injections with either human BMCs extract (hBMC) (n=6), human MNCs extract (hMNC) (n=8) or control with 0.5% BSA ( n=7) as previously described [13]. Each heart was injected at day 3 post-MI with the extract from 1×106 cells in 10 µl, or 10 µl BPBS/BSA, divided into two 5 µl injections into the anterior peri-infarct wall of the left ventricle.
TISSUE ANALYSIS
At day 28, the hearts were injected with a saturated solution of KCl (0.1 ml) to arrest in diastole. Heart samples were collected for histological staining. Scar size in the left ventricle and blood vessel density was analyzed for each group at day 28 post-MI as previously described [12]. Briefly, sections from the mid-ventricular level were examined for detection of infarct size, and blood vessel density using Masson’s trichrome-staining, and alpha-smooth muscle actin (SMA; Sigma-Aldrich, St. Louis, MO; 1:100 dilution), respectively. Blood vessel density was analyzed separately from the infarct zone, the border zone and the remote zone. Border zone was defined as an area containing one third of infarcted myocardium.
STATISTICAL ANALYSIS
Data were presented as mean ± SD. For the comparison of echocardiographic functional parameters and histological data (infarct size and blood vessel density) between groups, an ANOVA with Fisher’s post hoc test was used. For the comparison of LVEF changes at day 2 and day 28 within each group, paired t test was used. A p-value of <0.05 two-sided was considered significant.
RESULTS
Cardiac Function Over Time
After the induction of MI, all animals had an expected decrease in the left ventricular ejection fraction (LVEF) from 56.4 ± 2.6% to 36.7 ± 3.2% (p<0.001). After therapy, only the hBMC and hMNC cell extract groups resulted in improvement of the LVEF at day 28 post-MI (Figs. 1 and 2). The control group resulted in no cardiac functional benefit but rather continued worsening of LVEF over time, consistent with our prior report.12 hMNC extract resulted in significantly less ventricular remodeling post-MI as assessed by cardiac volumetric analysis and there was a trend for smaller ventricular volumes with the hBMCs compared to control (Fig. 3).
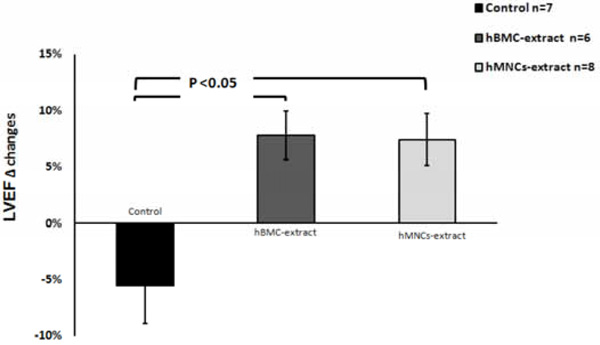
Echocardiographic changes in the left ventricular ejection fraction (LVEF) from day 2 to day 28 post-MI. Both human BMC and MNC extracts significantly improved % LVEF change compared with control which showed continued deterioration of the LVEF post-MI.
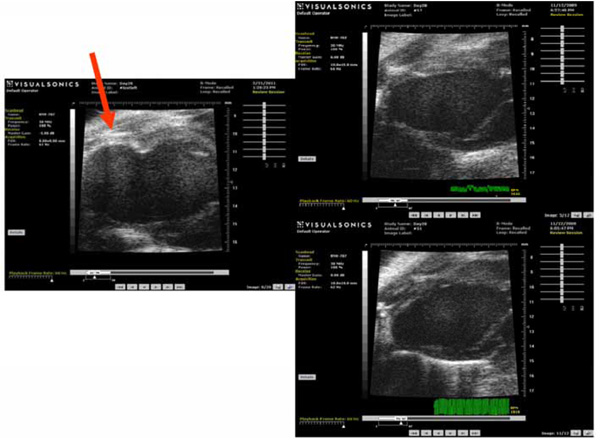
This figure demonstrates representative echocardiograms of the various groups: The left image shows an example of a remodeled heart from the control group with LV dilation and apical aneurysm (red arrow). Right upper panel is an example of a heart treated with hBMC-extract and the right lower panel shows an example of an animal treated with hMNCs-extract. Both of these demonstrate limitation of LV dilation and less geometric changes compared to the control group.
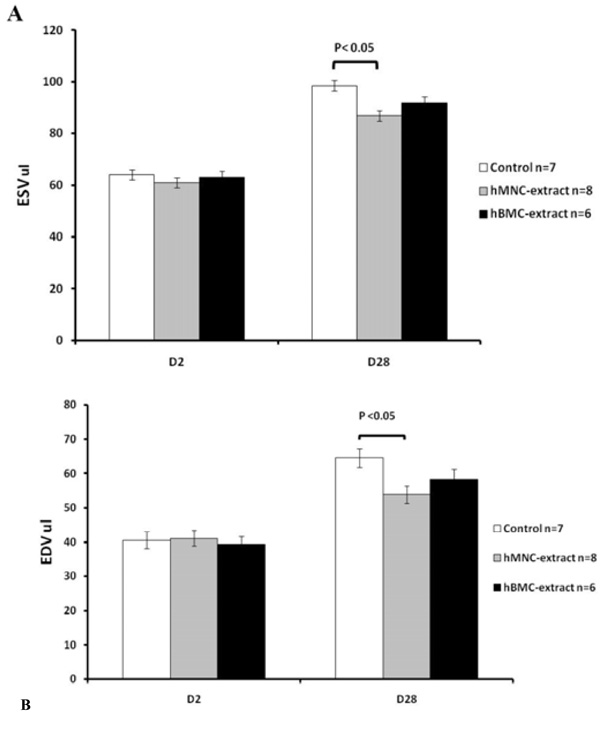
Changes in left ventricular volumes over time following myocardial infarction by echocardiography. (A) End systolic volume and (B) end diastolic volume at day 2 and at 4 weeks post myocardial infarction. Data are shown as mean ± SD. At day 2, pre-MI, all three groups have similar ESV and EDV values. hMNC extract was associated with significantly smaller volumes at day 28 compared to the control group and there was a trend towards smaller volumes with the hBMC extract but this did not reach statistical significance.
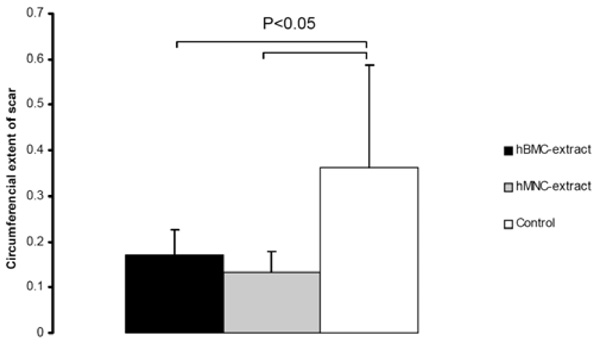
Human BMC and MNC extracts result in smaller infarct scar sizes compared to controls.
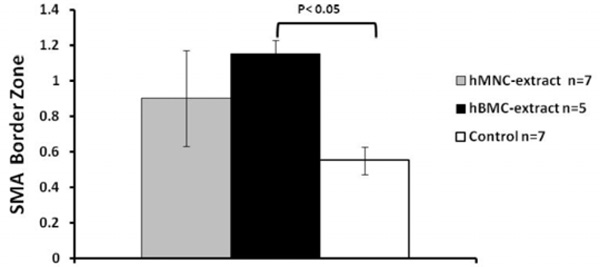
HMNC extract and hBMC extract had more vessels in the border zone than the control group but only the hBMC group reached statistical significance.
INFARCT SCAR
At day 28, both hBMC and hMNC extracts were associated with a significantly smaller infarct scar compared to the control group as shown in Fig. (4).
BLOOD VESSEL DENSITY EVALUATION
HMNC extract and hBMC extract had more vessels in the border zone than the control group but only the hBMC group reached statistical significance (Fig. 5). There was no difference in blood vessel counts in the infarct or remote zones (data not shown).
DISCUSSION
In this study, we have demonstrated that extracts derived from human BMC and MNC preserve cardiac function and decrease scar size post-MI when compared to the control group. Mouse and human bone marrow cells have several differences, which would potentially result in different outcomes as therapeutic agents [17-20]. Therefore, our results expand our previous report with the mouse BMC extract to human cells reinforcing that intact cells do not appear to be necessary to obtain cardiac benefit post-MI.
We and others have reported that mouse BMCs can aid in tissue preservation post-MI via a paracrine mechanism [12]. In our previous report, we demonstrated an early increase in vascularity at day 6 but at day 28 after injection of extracts derived from mouse BMC, this increase in vascularity had equilibrated back to the expected level in normal tissue, while the overall cardiac functional benefits appear to have lasted for a longer time period. We hypothesized that increased vascularity early after MI rescued ischemic cardiomyocytes from apoptosis, thereby salvaging myocardium. These results are consistent with prior studies by Schuleri et al., [21] who demonstrated that an early increase in myocardial perfusion resulted in longer term functional improvement. Indeed, these authors also show no increase in vessel number after 8 weeks.
In the current study, although only hBMC extract was noted to have more vessels in the border zone at day 28, both treatment groups resulted in improvement of cardiac function and led to a reduction in infarct size (Figs. 1-3). Potential reasons for this difference should be highlighted. First, this could be due to a small sample size which may have affected statistical power. Second, several paracrine factors and cytokines might be differentially expressed within the whole BMCs vs MNCs extract. The detailed mechanism(s) and which paracrine factor(s) within each extract lead(s) to cardiac functional improvement following therapy are not yet fully understood, and this continues to be an important area of investigation within the field. Lastly, we only assessed the vessel count at day 28 post MI and not at an earlier timepoint. As discussed previously, we and others have shown that increased vascularity early after MI rescues ischemic cardiomyocytes from apoptosis, thereby salvaging myocardium, and that increased vascularity was not necessarily found at a later timepoint (12, 21).
The mechanisms underlying the beneficial effects of BMC implantation into post-MI hearts are complex and controversial. Identification of cellular factors present in the lysates with anti-apoptotic and cardio-protective properties are of key interest in the search for effective clinical therapies and gain insight into the possible mechanisms of cardiac functional improvement.
Additionally, there is controversy in the cardiology field as to whether human BMCs or MNCs are more optimal cells for human clinical trials, and here we report that the extract from either was beneficial and that neither intact cell is necessary to result in cardiac functional benefit post-MI. The use of the cell extract rather than cells themselves is an important strategy in the development of novel therapies post-MI. Use of the extract eliminates the issues of cell rejection for allogeneic cells and negates the need for invasive bone marrow harvesting for autologous cell transplantation. The extract as a whole, or factors within the extract, can be given at the time of an acute coronary syndrome as an “off the shelf” medication at the time of coronary reperfusion. Which factor(s) within the human extract results in cardiac functional improvement is a topic of intense investigation at the present time.
Our study has several limitations. First, we did not assess vessel counts at an early timepoint post-MI. In addition, having human cell control groups would potentially allow us to confirm the non-superiority of live cells compared with the cell extracts. However, the use of human cells in a mouse model would require changing the animal model including: use of immunocompromised animals (to avoid cell rejection) and antibiotic therapy. Use of cell lysate (even from human derived source) negates the need for the use of immunocompromised animals or antibiotic therapy. For these reasons, the whole cell group was not included in the current study to avoid these confounding factors which would then make our model not as clinically translatable and impact our ability to correlate the current data with our previous findings using mouse BMC.
In conclusion, our results show for the first time that hBMC and hMNC extracts improve cardiac function post-MI in a mouse model. Further studies should evaluate the benefits of the cellular extract and identify the cellular factors present within the extract with cardio-protective properties.
ETHICAL APPROVAL
Ethical Approval; the present study was reviewed and approved by the Institutional Animal Care and Use Committee of the University of California, San Francisco.
CONFLICT OF INTEREST
The authors report no conflicts of interest. The authors alone are responsible for the content and writing of the paper. This work was supported in part by the UCSF Cardiac Stem Cell Foundation to Y.Y.
ACKNOWLEDGEMENT
Declared none.