All published articles of this journal are available on ScienceDirect.
Noncoronary Collateral Myocardial Blood Flow: The Human Heart’s Forgotten Blood Supply
Abstract
The “noncoronary collateral circulation” (NCCC) or “noncoronary collateral myocardial blood flow” (NCCMBF), reaches the heart through a micro-vascular network arising from the bronchial, esophageal, pericardial, diaphragmatic, and aortic arteries. The left and right internal thoracic arteries (ITAs) along with their collateral branches also serve as a source of NCCMBF-a feature seen in other mammals. Under certain circumstances the ITAs have a high potential for developing collateral branches. In the case of severe Leriche syndrome or with chronic obstruction of the abdominal aorta, the ITAs can serve as the main or even sole source of blood supply to the lower limbs. It is also possible for the ITAs to develop angiographically visible branches that directly connect with the coronary arteries. In ischemic conditions there is a functional, ischemia-reducing extracardiac coronary artery supply via natural ipsilateral ITA anastomosis. To date we know little about NCCMBF and its potential benefits in clinical applications, which makes this a challenging and intriguing field of research. This paper reviews all available data on noncoronary collateral blood supply to the human heart.
INTRODUCTION
Despite commonly held beliefs that the coronary arteries constitute the only source of myocardial perfusion, and that coronary collateral circulation (CCC) is the only blood supply that warrants ongoing attention [1, 2], there is adequate evidence to suggest that the human heart benefits from a small additional source of blood. Called “noncoronary collateral myocardial blood flow” (NCCMBF) or “noncoronary collateral circulation” (NCCC), it reaches the heart from the arteries of surrounding structures [3-9], and relative to CCC is seldom investigated. The left and right internal mammary arteries (ITAs), along with their collateral branches, are among these non-coronary sources [7-10]. This paper reviews the available data on non-coronary blood supply to the human heart.
The Nature of NCCMBF
Phylogenesis and comparative anatomy - Extra-coronary sources of blood supply exist in mammals other than man. This can be understood from a phylogenetic perspective. Gill-breathing vertebrates possess both coronary and extra-cardiac arteries that arise from a position homologous to the upper dorsal aorta. As the lungs replaced the gills, the heart migrated caudally from the cervical to the thoracic region. The site of origin of the coronary arteries also migrated caudally to draw closer to the source of oxygenated blood [11]. Along with the migrations of the heart and coronary arteries, extra-cardiac vessels shifted from the upper to lower part of the dorsal aorta. Hence, extra-cardiac sources of blood supply in mammals are reminiscent of the extra-cardiac system found in gill-breathing vertebrates [12]. A dual blood supply may be observed in dogs, where there is an anastomotic network between the right internal thoracic artery (RITA) and the right coronary artery (RCA) [13, 14]. This network reaches the heart by crossing the surface of the superior vena cava. Dual circulation is well developed in the rat heart; indeed, both right and left cardio-mediastinal arteries (CMAs) exist [14]. In most cases the right CMA arises from the RITA and less frequently from the carotid system, while the left CMA generally arises from the left internal thoracic artery (LITA) and in about 10% of the cases from the left subclavian artery and much less frequently from the first intercostal artery. The CMAs cross the chest from a lateral to medial position, traversing the anterior surfaces, respectively, of the right and left superior venae cavae—the latter usually present in the rat. Once they reach the heart, they divide into deep and superficial branches. The deep branches turn towards thoracic structures, establishing anastomoses with tracheobronchial and esophageal arteries, while the superficial branches divide into lateral, medial, and central sub-branches that perfuse the left and right atria, the conduction system, and the pulmonary veins. The nodal artery that perfuses the sino-atrial node usually arises directly from the RITA; thus, the conduction system of the rat is especially protected by both extra-coronary and coronary sources [14].
Human anatomy - In man, the vascular network of extracardiac arteries was first studied by Albrecht von Aller, who, in 1803, identified communications with the coronary arteries [15]. Some decades later, Langer, by injecting the coronary ostia, established that connections exist between the coronary arteries and the mediastinal, parietal pericardial, diaphragmatic, and hilar pulmonary arteries-he published his observations in 1880 [16]. In 1926, Woodfrood demonstrated connections between the aortic vasa vasorum and the coronary arteries [17]. Two years later Wearn confirmed that after injecting a colorant in the coronary ostia, the aortic vasa vasorum colored in the same way [18]. Robertson emphasized the importance of pericardial fat arteries in cases where there is obstruction of the coronary arteries. He described, as did the above-mentioned authors, the anastomosis between the coronary arteries, the small vessels of the aortic adventitia, and other vessels of the chest [19]. Further research on this was carried out by Gross [20], Monckberg [21], Spalteholz [22], Warburg [23] and Krasner [24]. In more recent years, studies performed by Brazier and Buckberg pursued the best method to achieve myocardial protection during open heart surgery and described the risk of cardioplegic solution wash-out due to noncoronary blood supply [4]. They determined that this blood supply could be attributed to “noncoronary collateral myocardial blood flow.” The anatomy was detailed by Hudson, Moritz and Wearn [6] as follows: (1) around the origin of the coronary arteries are micro-vascular arteries connecting to the aortic vasa vasorum and the small arteries of the aortic adventitia, mediastinum, diaphragm, pericardium, and pericardial fat; (2) there are some coronary branches in the pulmonary infundibulum that connect with the small arteries of the pericardial reflections situated around the main pulmonary artery, and with the small vessels of the pulmonary arteries and their peripheral branches, i.e., the mediastinal, bronchial, and fatty tissue arteries; (3) around the pulmonary veins is a network connecting with the bronchial, mediastinal, and pericardial arteries; (4) around the ostia of the superior and inferior vena cava is a network communicating with atrial branches of the right coronary artery, aortic, and pulmonary vasa vasorum; and (5) around the great vessels are small arterioles in the pericardial reflections [4]. In autopsy studies it was demonstrated that the surrounding organs contributed branches to the heart. It was possible to demonstrate the passage of injection mass from the coronary arteries into the vessels of surrounding structures, as well as the passage of injection mass from the thoracic branches of the aorta into the heart [25]. The most important thoracic branch establishing connections with the heart is the pericardiophrenic branch of the ITA (Fig. 1). Other branches, in order of prominence, are the anterior mediastinal, pericardial, and bronchial arteries, the superior and inferior phrenic arteries, and the intercostal and esophageal branches of the aorta [6]. The thoracic branch sources of noncoronary blood supply have also been mapped by Bloor and Liebow [3] (Fig. 2). Connections are more developed in older patients in accordance with the observation by Robertson [19] and Gross [20] that arterial branches to the pericardial fat increase with age. This may be explained by the increased probability of ischemic heart disease in older people and their requirement for compensatory blood supply mechanisms.
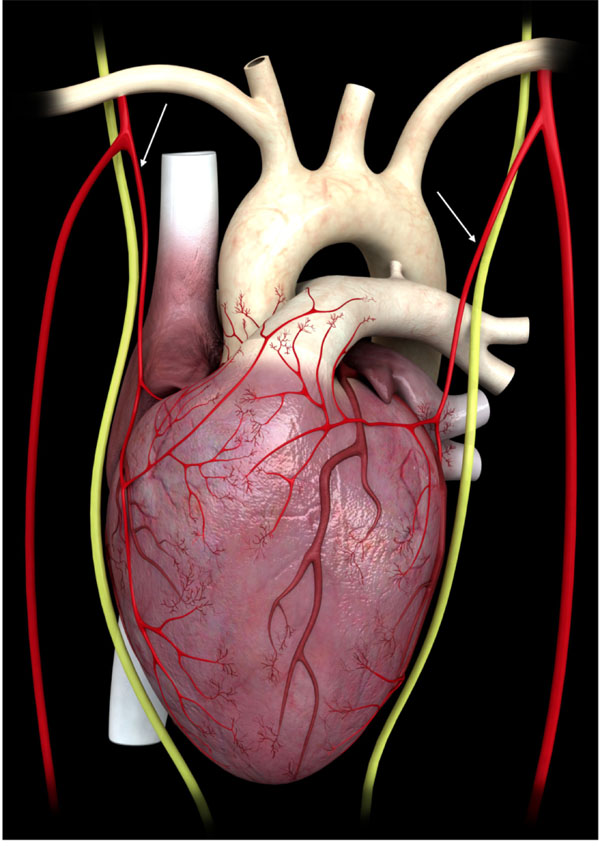
The internal mammary arteries give origin to the pericardiophrenic artery (arrows), which runs parallel to the phrenic nerve, through which it establishes connections with the heart.
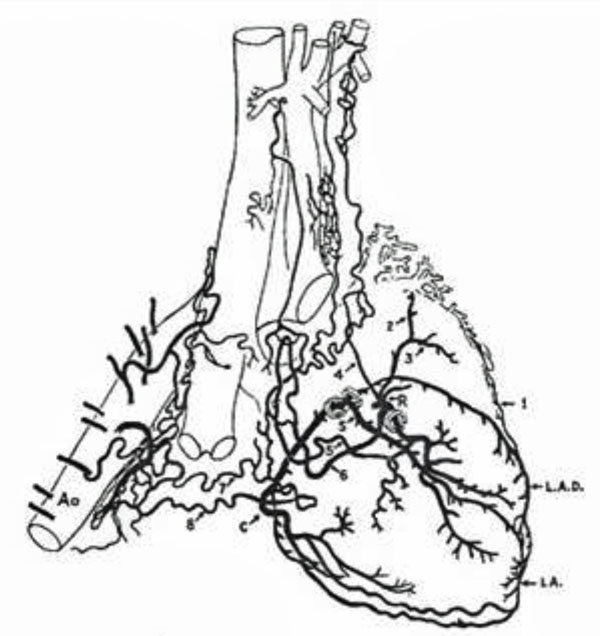
The non-coronary sources of blood supply to the heart come from heterogeneous thoracic arteries (reprinted with permission from Bloor CM, Liebow AA. Coronary collateral circulation. Am J Cardiol 1956; 238-52).
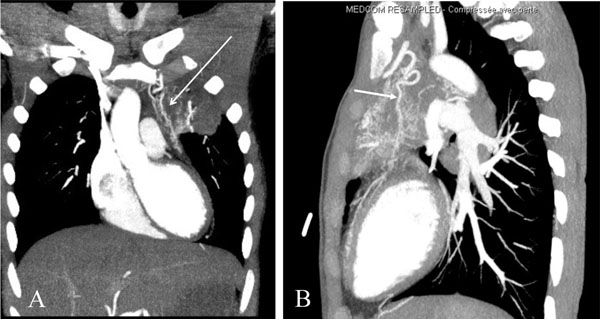
An angio CT scan showing a branch arising from the internal mammary artery directed to the heart in a patient affected by a pulmonary infection and ischemic heart disease. The artery is visible both in the frontal (A) and sagittal (B) planes (arrows).
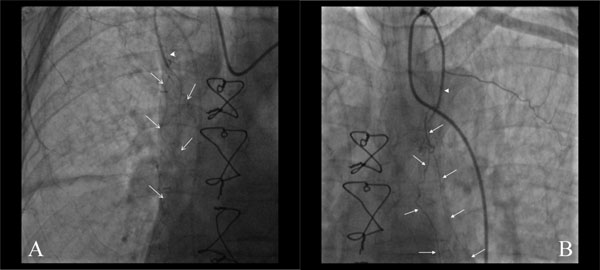
Selective angiography of the right (A) and left (B) internal mammary arteries in a patient who had undergone a CABG procedure 20 days earlier. The IMAs appear occluded (arrowheads), but in both cases fine new branches had developed from the patent segments, directed towards the heart (arrows).
Diameters, flow and physiology - The morphology of anastomosis may undergo change when pathologic conditions are present. Vessels can increase in caliber and become tortuous [10]. The diameters range from less than 1 mm up to 3 mm [25-30]. Vessels may reach a diameter of 2 mm in one out of eight patients [28, 29]-Kawasuji reported a vessel that reached 3 mm in diameter [30]. Nevertheless, even in patients with normal coronary arteries, anastomoses between the bronchial and atrial vessels of the coronary arteries are large enough to be seen by angiography. This important feature is said to exist in 20% of all humans [28].
Several investigators have attempted to estimate NCCMBF in dogs in the course of cardiopulmonary bypass using radioactive microspheres. Brazier and coworkers reported a flow rate ranging from 12 to 16 ml/100g/min [4]. They found that flow was greatest when the heart is arrested by aortic cross-clamping and in cases of ventricular hypertrophy. The authors suggested that NCCMBF might provide sufficient oxygen to the arrested left ventricle when metabolic needs are diminished due to hypothermic cardioplegia. Goldstein and associates found a mean NCCMBF rate of 3.4 +/- 0.16 ml/100 g/min following pharmacologic arrest with a range of from 8 to 261 ml/min [31]. In some patients the authors found no flow and attributed this to inaccuracies in the way the measurement was conducted. In a study by Baile et al., the NCCMBF ranged from 2.28 to 75 ml/min/100 g, with a mean value of 14 ml/min/100 g [32]. Coronary artery blood flow in normal resting patients has been reported to be 80+/-11 ml/min/100 g in one study [31] and 66+/25 ml/min/100 g in another [33]. So according to these measurements, NCCMBF is about one fifth that of coronary flow [32].
Noncoronary collateral vessels have the capacity to enlarge in response to pathologic conditions. The direction of flow depends on the conditions. In the case of supravalvular aortic stenosis, coronary arterial pressure increases and flow moves from the coronary arteries to the bronchial arteries [34]. This also occurs when bronchial arterial pressure decreases, as in Fallot tetralogy presenting pulmonary atresia [35] or stenosis [36]; or in the event of a pulmonary artery obstructive lesion, as is the case in Takayasu's arteritis, in which flow runs to the pulmonary circulation through the bronchial arteries [30]. Conversely, blood flow is directed to the heart when the pressure regimen is reduced in coronary arteries due to stenosis or occlusion [26, 28]. It has been reported that these anastomoses are more frequent in patients suffering from ischemic heart disease—being present in 50% of patients-rather than in people who do not suffer from ischemic heart disease, where they are present in 20% of the cases [28]. It has also been reported that the greater the severity of coronary stenosis, the greater the size of the anastomotic vessels [28]. There is further evidence of flow directed to the heart in angiographically documented cases of direct anastomoses between the ITAs and coronary arteries [37-41].
Observations from Clinical Practice
In cardiac surgery it is sometimes possible to observe blood pouring out of the coronary ostia while the aorta is open during aortic valve or ascending aorta procedures [4, 7, 8]. During CABG it is often possible to observe blood coming from the coronary arteriotomy. This can make the surgeon’s work more arduous due to reduced visibility at the anastomosis site [5, 7, 8]. This phenomenon may be observed despite proper cross-clamping of the ascending aorta and the positioning of a decompression line (vent).
Also, at times the heart does not cease beating or continues to fibrillate despite administration of a full dose of cardioplegic solution and adequate clamping of the aorta. Some angiographic scenarios point to additional conditions that imply the existence of a dual blood supply for the heart. The heart may continue to preserve good ventricular function despite the coexistence of a severely stenotic/occluded/ hypoplastic right coronary artery with a critical left main stem, or with total occlusion of two coronary arteries and sub-occlusion of a third [4, 42, 43]. If we acknowledge that under these circumstances blood supply from the coronary arteries is not sufficient to assure normal oxygenation and contractility, then preservation of an ejection fraction exceeding 55% can only be achieved through the existence of a secondary source of nourishment. Some rare autopsy studies on patients with chronic occlusion of three coronary arteries who died from non-cardiac causes indirectly support this assertion. Leary and Wearn reported on two autopsy cases having an intact endothelium and no thrombi in the coronary lumen, in spite of the apparent occlusion and hyalinization of the arteries due to a progressive chronic process [44]. Thorel reported the case of a man who died from carcinoma and whose coronary arteries at autopsy appeared to be obliterated proximally and over a long period of time. That patient presented extensive pericardial adhesions, and the heart had been nourished by neo-vessels existing in adherent scars [45]. These reports date from years ago, and prudent interpretation is advisable. However, it is a fact that the theory of neo-channel development within intrapericardial adhesions was adopted by many research surgeons of the pre-cardiopulmonary bypass era who were seeking a myocardial revascularization method. They created intra-pericardial adhesions in a number of ways; including injecting irritant agents in the pericardial cavity [46]; using skeletal muscle flap techniques [46-49]; and with cardio-omentopexy [46, 50], cardiopneumonopexy [46, 51, 52] cardiojejunopexy [46, 53], and gastrocardiopexy procedures [46, 54]. The results were variable, depending on the technique, but some of these procedures succeeded in supplying the heart through artificially created extra-coronary circulation channels. They did not limit themselves to animal experimentation models but also developed myocardial revascularization procedures for humans [46-49]. They had some success relieving patients’ angina or even returning them to work activities [47]. These outcomes demonstrated that, in cases of coronary insufficiency, the heart could be nourished through clinical improvements brought about by the development of artificially created noncoronary sources. Similarly, under ischemic conditions the heart may be nourished by naturally occurring extra-coronary blood supply sources. The latter would explain the good ventricular function seen in patients with almost total occlusion of all three coronary arteries, as well as the aforementioned phenomena observed during cardiac surgery. In this view, NCCMBF may serve as a compensatory mechanism in the presence of pathologic conditions. NCCMBF has been visually observed, presenting as an outpouring of blood from the coronary arteriotomy site in 16 of 58 patients - a total of 22 out of 168 coronary arteries were evaluated - affected by ischemic heart disease who had undergone CABG [5]. It was not merely a coincidence that this was observed more frequently in patients with triple-vessel disease (34%) and in patients presenting with, rather than without, (p<0.005) intercoronary collateral flow at angiography [5]. This suggests that the increase in NCCMBF provided an alternative blood supply during myocardial ischemia. In myocardial biopsies from dogs subjected to cardiopulmonary bypass and cardioplegic arrest, the presence of erythrocyte-filled blood vessels pointed to the possibility of direct access of NCCMBF to the heart, despite correct aortic cross-clamping [55]. Notwithstanding the compensatory effect of NCCMBF in ischemic heart disease, its role in cardiac surgery may be detrimental. Very few authors suggested that NCCMBF could reduce ischemic injury [55]. The majority believed NCCMBF could be detrimental because it can cause cardioplegic solution wash out, which in turn precipitates renewed electromechanical activity and the failure of perioperative heart protection [4, 5, 33, 34].
The ITAs’ Potential for Developing Collateral Branches
The ITAs each arise from the left and right subclavian arteries. They run in a cranio-caudal direction beneath the parietal pleura, 3 to 4 centimeters away from and parallel to the sternal border. The branches of the ITAs consist of the anterior intercostal, pericardiophrenic, perforating, muscolophrenic, and superior epigastric arteries [56-59]. The ITAs have the potential for developing collateral branches under some circumstances. It has been widely demonstrated that in cases with severe Leriche syndrome or with chronic obstruction of the abdominal aorta, the ITAs can evolve into the main or even sole source of blood to the lower limbs [60-68]. The latter occurs by virtue of the superior epigastric terminal branch, which shunts blood flow to the inferior epigastric arteries, which then anastomose with the external iliac arteries, eventually bypassing the aortic obstruction. It is also possible for the ITAs to develop angiographically visible branches that directly anastomose with the coronary arteries. This latter phenomenon has been demonstrated in several patients for both the right [37, 38] and left ITA [39-41]. Recently the author of this manuscript reported on a collateral branch arising from the left ITA directed towards the left anterior descending artery in a patient affected by a pulmonary infection and ischemic heart disease (Fig. 3A, B) [69]. Autopsy results from patients who died from ischemic heart disease confirmed this potential for plasticity. Similar connections were found in 12% of the cadavers observed [10]. The potential of the ITA to form collateral branches was also the basis for the Vineberg operation performed in the 1940s. It involved implanting the left and/or right ITA into the left ventricle free wall, leading to the development of an extensive vascular network in the myocardium and relief from angina [46, 70, 71]. This network was evident angiographically even at long-term follow-up [72, 73]. More recently (1993), Unger et al. confirmed in canine models that implanting ITAs in intramyocardial tunnels improved ITA-derived collateral blood flow, provided nutritive supply, and improved left ventricular function. Also, continuous infusion of heparin, rather than acid fibroblast growth factor, promoted new ITA-related collateral anastomoses [74-76]. Another example of the ITAs’ potential for collateralization was seen following imperfect coronary surgery, where blood flow was impeded by stenosis at the ITA-to-coronary artery anastomotic site, and eventually by graft occlusion in its middle-distal portion. In such a cases new branches arose from the ITAs and directed toward the heart within a few months of surgery [39]. However, this phenomena may be noticed even within a much shorter interval (Fig. 4A, B). These observations suggest that ITAs exhibit a significant potential for plasticity that may be induced under hypoxic conditions and/or in the presence of an obstruction to anterograde flow. Since ITA blood flow to the lower body increases with leg ischemia, one can speculate analogously that when blood flow is induced by a myocardial ischemic stimulus, it is preferentially diverted through branches directed to the heart rather than to the chest wall [7, 77, 78].
Initial studies on ITA-related NCCMBF - Prior to the first successful on-pump open heart operation by Gibbon in 1953 [79-81], and the global spread of coronary surgery in the 1960s and 1970s [46, 82], myocardial revascularization was achieved through ingenious and heterogeneous techniques [46-54, 83-85]. Early research in the US by Claude S. Beck demonstrated that the heart could be revascularized by neovessels developed within artificially created pericardial adhesions [46-49, 86, 87]. In Italy in 1939, Davide Fieschi performed a surgical ligature of the internal mammary arteries in one patient [88]. The patient experienced no angina at two years follow up. World War II impeded research in this field but Battezzati et al. [89] adopted these methods again in the 1950s. The rationale was that ligature of the ITA caused a local hypertensive status that resulted in an increase in perfusion pressure within the channels leading to the heart, principally the pericardiphrenic artery. Surgical access consisted of bilateral incisions of a few centimeters under local anesthesia between the second and the third ribs [89-94]. In 1959 Battezzati reported very encouraging results in the Am J Cardiology on 304 patients [95]. He saw symptomatic improvement in 94.8% of the patients and electrocardiogram improvement in 64.1%. The authors concluded that surgical ligation of the ITA produced “an increased blood supply to the myocardium through natural and pre-existing anastomotic channels” [95]. Follow-up ranged from 3 months to 4 years later. The clinical data confirmed what the same authors had proposed experimentally while working with canine models and human cadavers where they injected methylene blue into the mammary arteries and witnessed the onset of blue areas in the epicardium and in myocardial slices [89]. In Philadelphia, Glover demonstrated that ligation of the ITA prior to coronary artery occlusion prevented many dogs from dying, whereas all dogs died within 24 hours in the group in which coronary occlusion was not preceded by ITA ligation. Specifically, 58% of the dogs in the ligated group survived five days or more and 36% recovered completely. In humans, Glover’ data confirmed Battezzati’s encouraging results and the clinical value of the technique [96-98]. In 1958 Taber and Marchioro, working in canine models, observed a rise in subclavian artery pressure after ligation of the ITAs via an artificial-tubing system that replicated the ITA-pericardiophrenic artery system. They observed a rise of pressure in both arteries and concluded that blood flow through the pericardiophrenic artery may increase after ITA ligation [99]. Kitchell et al. reported that 68% of patients who had undergone ITA ligation found relief from angina [100]. Botham and associates, working with canine subjects, found that a consistent rise in pressure in the proximal segment of the ITA not only transmitted to its branches but also to the overall systemic and coronary arterial system. This suggested that such a generalized rise in pressure was due, in part, to neural and/or humoral factors [101]. This spurred a debate in the scientific community because other authors reported conflicting results. In 1959 and 1960, Cobb et al. [102] and Dimond et al. [103], respectively, also reported relief from angina in patients following a sham ITA ligation and so asserted that the placebo effect adequately explained the improvement in symptoms. However, just as the debate intensified the advent of cardiopulmonary bypass and on-pump coronary surgery relegated it to oblivion-that is until recently.
New studies on ITA-related NCCMBF - Since 2010, the author of this manuscript has sought to promote the principle of ITA occlusion through theoretical speculation based on a comprehensive reading of the historical record and more recent experimental work [7, 8, 49, 69, 77, 78, 104]. An experimental protocol was carried out in canine models. It involved LITA surgical occlusion, injection of vascular endothelial growth factor into the LITA, and the use of the neutron-activated microsphere technique. However, this first attempt in 50 years proved to be too onerous for the dogs, which were scheduled for three operations each [69]. In 2011, an anatomical report on this topic was published by Loukas et al. [9]. A very recent study by Stoller, De Marchi, and Seiler investigated the function of mammary-to-coronary artery anastomosis and its effects on myocardial ischemia in 120 patients [105]. This was done by calculating the collateral flow index (CFI) and intracoronary ECG ST segment elevation. The protocol involved induction of myocardial ischemia by coronary artery balloon occlusion with and without simultaneous distal ITA occlusion by angioplasty balloon. Complete occlusion of the ITA and the coronary artery was established by angiography. A total of 360 measurements were performed. The CFI was significantly higher in the presence versus the absence of IMA balloon occlusion (p<0.0001). Also, intracoronary ECG ST elevation was significantly reduced in the presence versus the absence of simultaneous ITA occlusion, and angina tended to diminish. By adopting modern tools this study confirmed the old theory that there is a functional, ischemia-reducing extracardiac coronary artery supply via natural ipsilateral ITA anastomosis [105].
NCCMBF and the Issue of ‘No-option’ Patients
Despite the improvement in coronary artery bypass grafting and percutaneous coronary intervention techniques, there is still a considerable number of patients not amenable to conventional myocardial revascularization due to the presence of diffuse coronary artery disease or severe co-morbidities, the so-called ‘no-option’ patients [106-109]. It is difficult to quantify the exact percentage of the population these patients represent but in daily practice this condition is encountered with increasing frequency due to the growth in the elderly population. It is estimated that in the United States alone, from 300,000 to 900,000 patients experience refractory angina pectoris, with some 25,000 to 75,000 new cases diagnosed each year [110]. Extrapolating, this means there are millions of patients worldwide suffering from angina pectoris whose only option is to receive medical therapy. Despite the advent of new drugs (e.g., Ranolazine [111], Ivabradine [112], and Nicorandil [113]) and the existence of non- pharmacologic treatments (e.g., transcutaneous electrical nerve stimulation [114], spinal cord stimulation [115], enhanced external counterpulsation [116], laser transmyocardial revascularization [117], extracorporeal shockwave myocardial revascularization [118]), the problems no-option patients face have not yet been resolved.
To date we know little about noncoronary blood supply to the heart and its potential benefits in clinical settings. That makes this both a challenging and intriguing topic for research. Aiming at enhancing the NCCMBF, there are many compelling arguments for pursuing research into the potential role of ITAs as an ipsilateral blood supply. Naturally this raises the question on how to achieve the therapeutic benefits.
Occlusion of ITAs has been successfully achieved in the past through surgical ligature, or more recently by experimental balloon inflation distal to the origin of the pericardiophrenic artery. Nowadays, occlusion of the mid-distal segments of the ITAs might be accomplished using an endovascular approach [77], i.e., embolization-a safe, low cost, and widely adopted endovascular procedure. It remains to be seen whether speculation along these lines can spur a new line of investigation that yields effective treatments for no-option patients.
CONFLICT OF INTEREST
The author confirms that this article content has no conflict of interest.
ACKNOWLEDGEMENTS
Declared none.