Statin-Induced Increase in HDL-C and Renal Function in Coronary Heart Disease Patients§
Abstract
Background: Little is known about the potential of statin-induced high-density lipoprotein cholesterol (HDL-C) increase to improve renal function in coronary heart disease (CHD) patients.
Methods and Results: In this post hoc analysis of the GREek Atorvastatin and Coronary heart disease Evaluation (GREACE) Study we investigated the effect of HDL-C increase after statin treatment on renal function. From a total of 1,600 patients, 880 were on various statins (mainly atorvastatin) and 720 were not. Other secondary prevention therapies were similar in the 2 groups. After a 3 year follow up, the lipid profile was unchanged in the statin untreated group and estimated glomerular filtration rate (eGFR) was reduced by 5.1% compared with baseline (P<0.0001). In contrast, in the statin treated group non-HDL-C was reduced by 43%, HDL-C was increased by 7% and there was a significant increase in eGFR compared with baseline by 9.8% (P<0.0001). In multiple regression analysis, the mean 7% increase in HDL-C in the treated arm during the entire study was associated with a 5.6% increase in eGFR recorded after the 6th week of treatment. The odds ratio of eGFR increase with every 5% statin-induced rise in HDL-C was 1.78 (95% confidence interval 1.19-3.34; P=0.001).
Conclusions: Statin treatment significantly improved renal function. Statin-induced HDL-C increase significantly and independently contributed to this improvement. This finding supports the concept that improving lipid variables other than low density lipoprotein cholesterol is also beneficial to preserving renal function.
INTRODUCTION
Chronic kidney disease (CKD), including mild renal impairment, is recognized as an independent predictor of cardiovascular disease (CVD) in high-risk patients [1–3]. Similarly, in the general population there seems to be a graded association between a reduced glomerular filtration rate (GFR) and the risk of death, CVD events and hospitalization [4].
Dyslipidaemia is an independent risk factor for both CVD and CKD. The Physicians’ Health Study reported that from 4,483 participating healthy men with a normal baseline renal function, those with low high-density lipoprotein cholesterol (HDL-C) and high non-HDL-C [low-density lipopro-tein cholesterol (LDL-C) + very LDL-C (VLDL-C) + intermediate density lipoprotein cholesterol (IDL-C)] levels had double the risk for CKD after adjusting for other risk factors [5]. A recent study also showed that high apolipoprotein B and non-HDL-C levels were associated with an increased risk for deterioration of renal function in patients with CKD [6]. The issue is whether or not hypolipidaemic drug treatment is able to reverse this process.
The results of the Helsinki Heart Study [7] and the Veterans Affairs High-Density Lipoprotein Intervention Trial (VA-HIT) [8] showed that fibrates might not exert a clinically relevant effect on rates of kidney function loss in individuals with low HDL-C and elevated non-HDL-C. The Heart Protection Study (HPS) showed that allocation to sim-vastatin significantly attenuated the fall in estimated-GFR (eGFR) compared with placebo [9]. A pooled analysis of 3 pravastatin survival trials also showed a decreased deterioration of renal function with pravastatin compared with placebo [10]. In the GREek Atorvastatin and Coronary heart disease Evaluation (GREACE) Study, statin treatment significantly increased eGFR by 12% and reduced serum uric acid levels, whereas renal function deteriorated in usual care patients [11–13]. Of note, the GREACE trial [11–13] showed that statin-induced improvement of eGFR was more evident in patients with mild renal impairment and contributed to the reduction in CVD events (multivariate analysis). A post hoc analysis of the Treating to New Targets (TNT) trial showed that over the 5 year study period there was a significant by 5.6% and 8.4% increase in eGFR with both doses of atorvas-tatin (10 and 80 mg/day, respectively) instead of the expected decline of about 5 mL/min/1.73 m2 [14].
However, it is not yet clear whether the relatively small increases in HDL-C observed during statin therapy contribute to the improvement in renal function, beyond the established beneficial effect of LDL-C reduction and non-HDL-C. In the present post hoc analysis of GREACE we address this issue.
STUDY POPULATION - METHODS
Study design, patients and methods. The design of the GREACE study and its main findings have been previously described [11,12,15,16]. Briefly, GREACE included men (78%) and women (22%) with established coronary heart disease (CHD), aged <75 years (mean 58.3 years). Their serum LDL-C concentration was >100 mg/dL (2.6 mmol/L) and serum triglyceride (TG) levels <400 mg/dL (4.5 mmol/L). All patients attended the Atherosclerosis or the Metabolic Syndrome Units of the Hippocration University Hospital, Thessaloniki, and if eligible were randomized either into the structured care group, followed up by the University Clinic, or into the usual care group followed up by heart specialists or general practitioners of the patient’s choice outside the hospital. In the structured care group, the starting dose of atorvastatin was 10 mg/day. With evaluations every 6 weeks the dose of atorvastatin was titrated up to 80 mg/day for patients not reaching the National Cholesterol Education Program Adult Treatment Panel III (NCEP ATP III) LDL-C goal <100 mg/dL (2.6 mmol/L) with lower dosages. Patients in the usual care group were treated according to their physician’s standard of care. Usual care consisted of lifestyle changes plus necessary drug treatment, including lipid-lowering agents. Atorvastatin was not excluded from the usual care group. After dose titration patients were followed for a mean 3 year period with visits every 6 months. Serum creatinine (SCr) was measured using the Jaffé method (Olympus Diagnostica GmbH, Clare, Ire-land); normal range 0.6-1.3 mg/dL (55-115 μmol/L). eGFR was calculated using the Modification of Diet in Renal Disease (MDRD) formula [17].
The study subjects were randomized either to structured care (n=800) with dose titration of atorvastatin to achieve the LDL-C target of <100 mg/dl (2.6 mmol/L) or to usual care (n=800) that was followed by physicians of their choice. In this later group only 12% of subjects were on statin treatment and only 3% were at LDL-C target. In the present post hoc analysis we divided participants into those on statin and those without statin treatment, irrespective to their initial allocation to structured or usual care groups. Patients with SCr levels above the reference range [>1.3 mg/dL (115 μmol/L)] were excluded from the study.
Classification of CKD. In the Kidney Disease Outcomes and Quality Initiative (K/DOQI) guidelines [3], CKD is defined according to the presence or absence of kidney damage and level of kidney function, irrespective of diagnosis. In this classification scheme including 5 stages, stage 1 is associated with a normal eGFR (≥90 ml/min/1.73 m2), stage 2 with kidney damage and mildly decreased eGFR (60-89 ml/min/1.73 m2), stage 3 with moderately decreased eGFR (30-59 ml/min/1.73 m2), stage 4 with severely decreased eGFR (15-29 ml/min/1.73 m2) and stage 5 with an eGFR < 15 ml/min/1.73 m2.
Statistical Analyses. Treatment-based analyses of all patients according to statin or no statin treatment were performed. On study eGFR values were compared with those at baseline using ANOVA to assess differences over time within and between treatment groups. A univariate analysis was initially performed, including 25 predictors of renal function deterioration. Then, after removal of 6 predictors with a P > 0.10, 19 predictors were included in a multivariate Cox Predictive Model, involving backward stepwise logistic regression. All predictors were recorded as categorical factors (0-1). All univariate or multivariate analyses were performed with an HDL-C by 4 mg/dl (0.1 mmol/L) stepwise increase or reduction from baseline. Data were expressed as mean values. Standard deviations are presented in both Tables 1 and 2. The SPSS 11.01 software package (SPSS, Inc., Chicago, IL) was used for all statistical analyses. A two-tailed P < 0.05 was considered significant.
Baseline Characteristics of the Statin Treated and Untreated Groups
Parameter | Statin Treated (N=880) | Statin Untreated (N=7200) | P Value |
---|---|---|---|
Men/women (%) | 79/21 | 78/22 | NS |
Age (years) | 59±9 | 59±13 | NS |
CHD (%) | 100 | 100 | NS |
Dyslipidaemia (%) | 100 | 100 | NS |
Current smokers (%) | 4.4 | 3.7 | NS |
History of diabetes mellitus (%) | 20 | 19 | NS |
History of arterial hypertension (%) | 43 | 42 | NS |
Congestive heart failure (%) | 8 | 7 | NS |
Prior PCI/CABG (%) | 35 | 36 | NS |
Recent hospitalization for CHD (%) | 7 | 8 | NS |
Renal function K/DOQI | |||
Stage 1 (%) | 41 | 40 | NS |
Stage 2 (%) | 54 | 53 | NS |
Stage 3 (%) | 5 | 7 | NS |
NS: not significant.
CHD: coronary heart disease, PCI: percutaneous coronary intervention, CABG: coronary artery bypass graft, K/DOQI: Kidney Disease Outcomes and Quality Initiative.
Lipid Parameters (Mean Value ± Standard Deviation) at Baseline and During the Study in the Statin Treated and Untreated Groups
Lipid Parameter | Statin Treated (n=880) | Statin Untreated (n=720) | Differences Between On-Study Values | ||
---|---|---|---|---|---|
Baseline Value | Mean On-Study Value & % Change vs Baseline | Baseline Value | Mean On-Study Value & % Change vs Baseline | Statin vs No Statin P Value | |
Total cholesterol (mg/dl) | 258±35 | 164±9 -36%† | 256±39 | 244±40 -4% | <0.0001 |
LDL cholesterol (mg/dl) | 181±23 | 99±3 -46%† | 180±26 | 175±24 -3% | <0.0001 |
VLDL cholesterol (mg/dl) | 37±11 | 24±5 -31%† | 36±9 | 35±10 -2% | <0.0001 |
HDL cholesterol (mg/dl) | 39±6 | 42±5 7% * | 39±7 | 40±6 1% | 0.002 |
Non-HDL cholesterol (mg/dl) | 219±32 | 125±6 - 43%† | 217±29 | 206±31 -5% | <0.0001 |
Triglycerides (mg/dl) | 182±61 | 127±31 -30%† | 179±59 | 173±64 -4% | <0.0001 |
† P< 0.0001 vs baseline.
* P< 0.01 vs baseline.
LDL: low density lipoprotein, VLDL: very low density lipoprotein, HDL: high density lipoprotein
To convert data from mg/dL to mmol/L divide total cholesterol, LDL cholesterol, VLDL cholesterol, HDL and non-HDL cholesterol values by 38.7; triglyceride values by 88.5.
Medical Therapy During the Study
Statin Treated N=880 | Statin Untreated N=720 | P Value | |
---|---|---|---|
Aspirin or other antiplatelet agents (%) | 87 | 86 | NS |
Beta-blockers (%) | 85 | 84 | NS |
ACE inhibitors or ARBs (%) | 54 | 53 | NS |
Nitrates (%) | 14 | 15 | NS |
Calcium channel blockers (%) | 26 | 27 | NS |
Diuretics (%) | 11 | 12 | NS |
Statins (%) | 100 | 0 | < 0.0001 |
NS: not significant.
ACE: angiotensin converting enzyme, ARBs: Angiotensin II receptor blockers.
Univariate Odds Ratios and 95% Confidence Intervals for eGFR Increase After the 6thTreatment Week
Variables | Univariate ORs and 95% CI for eGFR Increase | |
---|---|---|
OR (95% CI) | P Value | |
Age (years) | 0.47 (0.34-0.64) | <0.0001 |
Gender male | 1.44 (1.07-1.93) | 0.015 |
CVD event during the study | 0.82 (0.65-1.17) | 0.2 |
Use of beta-blockers during the study | 0.67 (0.50-0.91) | 0.02 |
Use of ACE inhibitors during the study | 1.27 (0.95-1.02) | 0.064 |
*On treatment non-HDL-C <130 mg/dL | 5.60 (4.00-7.85) | <0.0001 |
HDL-C with every 5% increase | 3.49 (2.53-4.82) | <0.0001 |
Fourteen univariate predictors of all CHD-related events all with p <0.10 (the above plus: current smoking, family history of premature CHD, history of hypertension, history of diabetes mellitus, prior revascularization, acute coronary syndrome, and prior myocardial infarction) were initially entered.
* On treatment values were considered as the mean values during the entire study.
To convert data from mg/dL to mmol/L divide non-HDL-C values by 38.7
OR: odds ratio, CI: confidence interval, CVD: cardiovascular disease, ACE: angiotensin converting enzyme, HDL-C: high density lipoprotein cholesterol.
Multivariate Cox Predictive Model for eGFR Increase After the 6th Week of Treatment (Backward Stepwise Logistic Re- gression)
Variables | Multivariate Cox Predictive Model for eGFR increase | |
---|---|---|
OR (95% CI) | P value | |
Age (years) | 0.79 (0.62-0.93) | 0.02 |
Gender male | 1.32 (1.14-1.65) | 0.003 |
CVD event during the study | 0.86 (0.64-1.37) | 0.4 |
Use of beta-blockers during the study | 0.82 (0.61-1.19) | 0.2 |
Use of ACE inhibitors during the study | 1.14 (0.84-1.04) | 0.1 |
*On treatment non-HDL-C <130 mg/dL | 3.12 (1.79-5.12) | <0.0001 |
HDL-C with every 5% increase | 1.78 (1.19-3.34) | 0.001 |
Fourteen univariate predictors of all CHD-related events all with p <0.10 (the above plus: current smoking, family history of premature CHD, history of hypertension, history of diabetes mellitus, prior revascularization, acute coronary syndrome, and prior myocardial infarction) were initially entered.
* On treatment values were considered as the mean values during the entire study.
To convert data from mg/dL to mmol/L divide non-HDL-C values by 38.7
OR: odds ratio, CI: confidence interval, CVD: cardiovascular disease, ACE: angiotensin converting enzyme, HDL-C: high density lipoprotein cholesterol.
RESULTS
Baseline characteristics of patients are shown in Table 1.
1. Renal Function at Baseline
According to K/DOQI, 642 patients had a stage 1, 864 a stage 2, and 94 a stage 3 renal function status. Patients from each renal function status were similarly distributed in the 2 analysed groups (Table 1). At baseline, eGFR in both analysed groups [with (n=880) or without (n=720) statin treatment] was 72 mL/min/1.73 m2 and 73 mL/min/1.73 m2, respectively (P = not significant).
2. Effect of Dyslipidaemia and Statin Treatment on eGFR
A. No statin treatment (n=720). The 88% of the usual care group and those on atorvastatin that could not tolerate the drug formed this control group. There was a significant by 5.1% reduction in eGFR (P<0.0001 vs baseline) at the end of the 3 year period (Fig. 1).
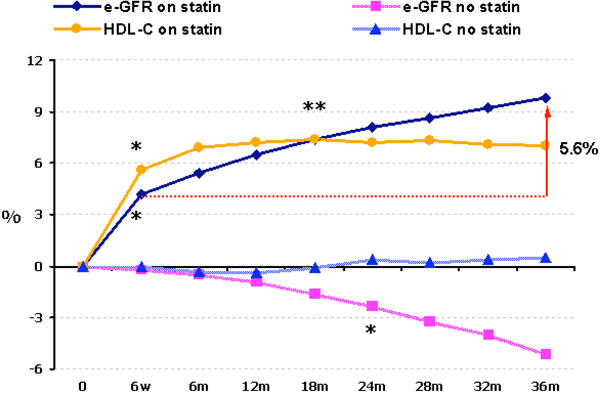
Time-course of changes of HDL-C and eGFR in statin treated and in statin untreated patients.
* P<0.0001 vs baseline.
**P<0.0001 vs 6th treatment week.
B. Statin treatment (n=880). From patients allocated to a statin, 808 were on atorvastatin (mean dose 23 mg/day), 41 were on simvastatin (mean dose 20 mg/day), 23 on pravas-tatin (mean dose 24 mg/day) and 8 on fluvastatin (mean dose 40 mg/day). These patients presented an increase in eGFR levels by 4.2% at the 6th week of treatment (P<0.001 vs baseline) and by 9.8% at the end of the study (P<0.0001 vs both baseline and 6th week of treatment) (Fig. 1). The net increase in eGFR between 6th week of treatment and the 3rd year of treatment was 5.6% (Fig. 1). This increase was dependent on baseline eGFR levels. Patients with a eGFR < 77 mL/min/1.73 m2 (median value of all patients) had a mean increase in eGFR of 12.9% (P<0.0001), while patients with an eGFR > 77 mL/min/1.73 m2 had a mean increase in eGFR of 4.5% (P=0.002). Thus, the greatest benefit from statin treatment was observed in those with early renal dysfunction.
3. Lipid Profile at Baseline
Patients from both treatment groups had high total cholesterol, LDL-C, non-HDL-C and TG levels (Table 2). HDL-C was 39-40 mg/dl (1.0 mmol/l). Thus, the dyslipidaemia of the GREACE study could be characterized mostly as combined hyperlipidaemia.
4. Effect of Statin Treatment on the Lipid Profile
The lipid profile in the untreated group remained essentially unchanged throughout the study (Table 2). In the statin treated group, 97% of patients (n=854) had mean LDL-C levels < 100 mg/dL (2.6 mmol/L) after the titration period (mean change -46%) and 98% of patients (n=898) had mean non-HDL-C levels < 130 mg/dL (3.4 mmol/L) after the titration period (mean change -43%). The mean increase in HDL-C was 7% (P<0.001 vs baseline and P=0.002 vs on-study values in the usual care group). The LDL-C/HDL-C ratio was reduced by 49% (P<0.0001 vs both baseline and usual care), while the mean reduction in TGs was 30% (P<0.0001 vs baseline).
5. Effect of HDL-C Increase on eGFR
Multiple regression analysis showed that the renal function improvement (estimated by eGFR increase) in patients receiving statins was associated with a reduction in athero-genic lipoprotein levels as well as with the statin-induced increases in HDL-C (7% from baseline), over and above secondary CVD prevention therapies. This effect of HDL-C was seen in patients who achieved LDL-C levels <100 mg/dl (2.6 mmol/L) and non-HDL-C levels <130 mg/dl (3.36 mmol/L).
6. Other Factors that may have Influenced the Improvement in Renal Function
There wre no significant differences between the statin treated and untreated groups regarding demographic characteristics, CHD risk factors at baseline (Table 1) and concomitant drug treatment (especially in angiotensin converting enzyme inhibitors or calcium channel blockers that might influence eGFR) (Table 3). The levels of glycaemic control and both systolic and diastolic blood pressure during the study were similar between the 2 groups. Both at entry and during the study, smokers (4.4% and 3.7%, respectively) were similarly distributed in the 2 treatment groups. Similar numbers of patients lost >10% of their body weight in the 2 treatment groups (40 in the statin treated and 44 in the untreated group). Furthermore, the MDRD formula considers age, gender and race. Thus, it was highly unlikely that we had false low or false high eGFR values. Moreover, results were fully adjusted for 20 predictors of CHD related events (Tables 4 and 5). Thus, the beneficial effect on renal function should mainly be attributed to statin treatment. There were no extreme values of SCr at baseline [i.e. extremely high values, because patients were excluded from the study if they had a SCr value >1.3 mg/dl (115 μmol/l)]. The method used to assess SCr is reproducible and the use of mean values of a large number of patients together with the different effects on SCr in each group reduced to a minimum any regression to the mean effect. Moreover, there was no relation between HDL-C and serum uric acid levels if eGFR was taken into consideration. All patients in both treatment groups received advice on life-style changes and the body mass index (a rough index of compliance with life-style measures) was similar in both groups.
DISCUSSION
We showd that renal function (represented by the eGFR) declines over 3 years in dyslipidaemic CHD patients with normal or mildly impaired renal function at baseline when they are not being treated with a statin. Statin treatment (mainly atorvastatin) not only inhibited this deterioration but significantly increased the eGFR in these patients. It has been proposed that CVD and renal disease progression are the consequences of the same underlying disorders [18]. Thus, CVD risk factor reduction strategies may also be beneficial in CKD [18,19]. Whether there are differences between statins in terms of renal benefit remains to be established [20].
In our tatin untreated patients, there was a gradual reduction in eGFR that became significant by the end of the 2nd year of the study; renal function continued to deteriorate until the end of the study (a mean 3 year period). In statin treated patients, renal function improvement was manifest as early as the 6th week of treatment; thereafter there was further improvement that continued until the end of the study. The early part of renal function improvement might be attributed to the pleiotropic effects of statins (e.g. anti-inflammatory effects and improved endothelial function) [21]. In support of these findings, when patients with CKD were given statins for CVD risk reduction, they showed evidence of improved renal function [22]. The improvement of renal function after the 6th week of treatment might be related both to reduction of atherogenic lipoproteins and the increase of HDL-C.
High seum LDL-C has an adverse effect on glomerular mesangial cells and endothelial cells [23]. Moreover, in animal studies [24], elevated lipid content of VLDL and IDL played an important role in the pathogenesis of proteinuria and glomerulosclerosis. Clinical and experimental studies have demonstrated the role of lipoproteins in the decline of renal function with emphasis on glomerulosclerosis [25–28], neutrophil and macrophage infiltration [29] and upregulation of the cytokine interleukin (IL)-6 [30,31] or endothelial nitric oxide synthase [32]. Animal studies showed that the rate of decline of GFR is beneficially modified after improving the lipoprotein profile by dietary or pharmacological manipulation, including statins [28,33].
The preent study addressed whether or not the statin-induced HDL-C increase contributed to renal function improvement on top of LDL-C reduction and other secondary CVD prevention measures. HDL might improve renal func tion through several mechanisms [34]. Reverse cholesterol transport may contribute to the inhibition of intra-renal atherosclerosis and protect against direct toxic effects of lipids on renal cells [35]. Inhibiting the accumulation of lipopro-teins may also reduce LDL binding to receptors expressed by the mesangial cells and limit matrix production [36]. Another relevant mechanism might be the antioxidant effect of HDL [37]. However, can HDL particles protect from atherosclerosis or glomerulosclerosis in both low and high LDL-C states? Our data suggest that they can. It is also plausible that low LDL-C and anti-inflammatory effects of statins might improve HDL particle performance [39]. First, lower concentrations of LDL-C leave less cholesterol to be “scavenged” by HDL particles. Second, lowering oxidized LDL levels results in inhibition of growth factor receptor activation and of subsequent matrix metalloproteinase upregulation [37,38]. Another mechanism of action of HDL results from the inhibition of the production, even at low LDL-C concentrations, of cellular reactive oxygen species (ROS) [37].
In the bsence of inflammation, HDL has a complement of antioxidant enzymes that work to maintain an anti-inflammatory state [39,40]. However, in the presence of systemic inflammation, these antioxidant enzymes can be inactivated and HDL can accumulate oxidized lipids and proteins that transform it to a proinflammatory particle [40]. This is probably the case for our statin untreated CVD patients.
Finally patients on statin treatment had fewer recurrent CVD events during the study, thus preserving cardiac performance and renal blood flow.
STUDY LIMITATIONS
The stuy has all the restrictions of a post hoc analysis and there was no assessment of urine protein. In addition, we did not directly measure GFR; an effect of statins on creatin-ine secretion from renal tubular cells cannot be ruled out and might have affected eGFR without an actual effect on renal function.
CONCLUSIONS
There ws a decline in renal function over a period of 3 years in CHD patients not treated with statins. In contrast, statin treatment significantly improved renal function. There was a contribution of statin-induced HDL-C increase (7%) to this improvement over and above low non-HDL-C levels and other potentially beneficial therapies. This evidence supports the concept that improving lipid variables other than LDL-C may be relevant for both CHD and CKD prevention.
DECLARATION OF INTEREST
The preent study was conducted independently; no Company or Institution supported it financially. Some of the authors have attended conferences and participated in advisory boards and other trials sponsored by various pharmaceutical companies.
NOTES
§ A post-hoc subgroup analysis of the GREek Atorvastatin and Coronary-heart-disease Evaluation (GREACE) Study.